An Overview of Battery Safety Methods
Batteries are at the heart of our energy and mobility future. If the recent supply chain crunch has shown us anything, it’s that batteries are even more successful than the most optimistic analyst predictions just a few years ago. But with the success of batteries and ever-increasing numbers of deployed battery packs around the world, we also see more incidents of failing systems – from burning electric scooters in apartment buildings in New York City to a series of electric bus fires in Paris and London.
While everyone in the industry agrees that battery safety should be the top priority, the reality is that the expectations and pressures relating to growth create conflicting priorities, in addition to the pressures to commercialize new, innovative technologies. Higher energy densities, for example, inevitably mean more energy that can fuel the fire during a failure. So, what are our options to prevent critical failures and make batteries—and clean energy—as safe as possible?
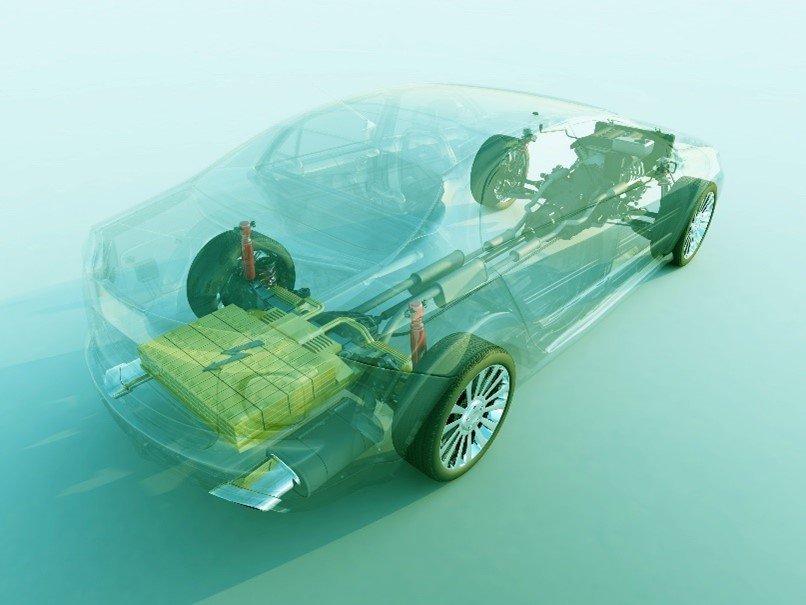
Faults and Financials
First, let’s examine the issue. How likely are electric vehicle (EV) fires due to a faulty battery and what does it mean financially? There are multiple reports on the issue of EV fires, including data released by Tesla in 2021 showing an average of one EV fire for every 205 million miles driven in the U.S. from 2012 to 2020. According to the U.S. National Fire Protection Association (NFPA), in the years 2018, 2019 and 2020 there was, on average, one vehicle fire per 17.8 million miles driven on U.S. highways. This suggests that vehicle fires occur more than ten times frequently in internal combustion engine (ICE) vehicles than in electric cars. We need to, however, also take into consideration that the average age of EVs is much lower than that of ICE vehicles. This means that we’re at different points in the respective bathtub curves and the occurrence of fires might somewhat equalize down the line.
The financial risks associated with faulty EV batteries can be derived from two major recalls that happened after a series of battery fires in 2020 and 2021: the Chevrolet Bolt (at least 17 incidents) and the and Hyundai Kona (at least 15 incidents). Both companies used LG batteries and the affected vehicles were produced between 2017 and 2020. In all cases, the batteries went into thermal runaway during or after charging. There were no known external reasons for the fires.
Interestingly, both Hyundai and Chevrolet first tried to fix the battery issue with a software update for the battery management system (more on this later). When an updated Kona EV caught fire in January 2021, Hyundai issued a general recall of all its 76,000 Kona electric vehicles and 6,000 IONIQ models and electric buses that use battery cells made by LG Chem. The recall ended up costing Hyundai US$900 million.
General Motors (GM) tried two iterations of software updates but was unable to prevent more battery fires, among others in Virginia and Vermont, U.S. After that, GM told customers to park their EVs outside and not charge them unattended, before finally issuing a formal recall a week later. The recall became the most expensive recall ever on a per-vehicle basis and eventually led LG to compensate GM over US$1.9 billion.
Three Options for Safer Batteries
So, what are the options that engineers have to make batteries safer and to reduce financial risk? Generally speaking, there are three main areas:
- Production quality
- System design
- Cloud analytics
1. Production quality
To operate safely, batteries need to be produced with the utmost care and precision, from processing active materials to manufacturing the cells to assembling the pack. Any production defect can lead to unexpected (and sometimes critical) behaviour years later.
The only solution to control manufacturing defects is rigorous quality management – so tighten your six-sigma black belt and dive into everything from incoming goods control to end of line testing. But there are two major challenges: for one, most mid-stream companies in the EV space have neither the full information about, nor the ability to impact the quality of the cells and packs they buy. On the contrary, in today’s market, the sheer ability to buy batteries from a supplier weighs heavier than any quality management (QM) certification.
On top of that, even the most rigorous quality management will never catch 100% of the failures. And at the giga-scale, even the tiniest slips become real problems. To reiterate using the recently announced Volkswagen Gigafactory as an example: a failure that only happens once in a million will happen at least five times every month in this factory.
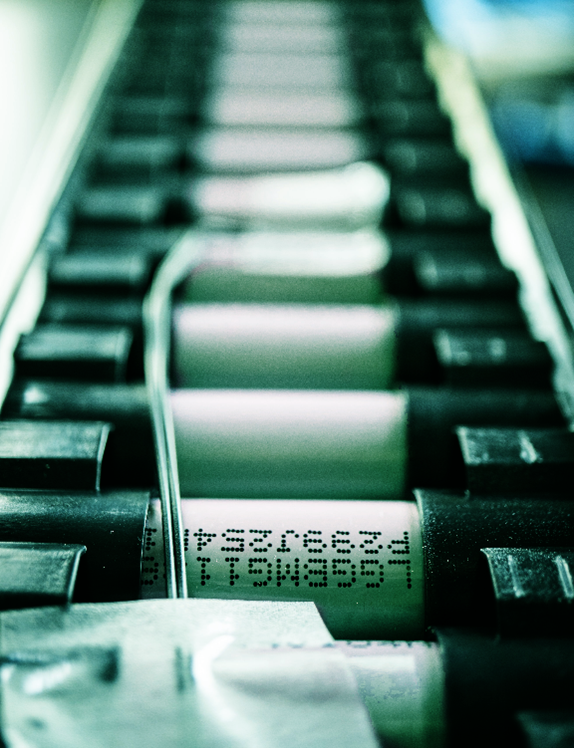
2. System design
Battery systems are equipped with several layers of protection. These protective measures are meant to keep the battery in its intended window of operation, shield it from external harm, and minimize the impact of a single-cell failure.
Passive safety components: lithium-ion battery (LIB) systems usually contain several passive components to ensure the safety of the overall system – from sturdy packaging to withstand crashes to hermetic sealing against water inflow. However, passive safety components are last resorts to minimize the damage from critical situations that are already happening. They generally cannot prevent safety incidents.
Battery management system (BMS): BMS’s are the brain of every lithium-ion battery. They make sure a cell is not over- or undercharged and they bring basic state estimation functionalities with them, including state of charge (SOC) and state of health (SOH).
However, BMS’s also have noticeable shortcomings: they only see the cells within the corresponding battery pack, have little to no access to historic data or data from other battery systems, and they have limited computing power. Due to these limitations, BMS capabilities to detect edge-case anomalies, run deep analytics robust against measuring errors (which happen all the time), and predict long-term trends are slim to none.
The limitation of BMS safety algorithms was highly visible in the attempts of Hyundai and GM to solve their safety problems with a firmware update in other words a BMS software update). While it’s possible to locally scan the sensor data for anomalies, such as rapidly changing impedances or sudden voltage drops, as precursors for thermal events, the lack of baseline data and ongoing comparisons to similar systems strongly limits the value of such analyses.
3. Cloud-based battery analytics
A proven strategy to improve battery safety is the use of cloud-based analytics. By detecting critical faults at an early stage using more sophisticated and modern analytical methods than available in any BMS, automobile manufacturers and electric car owners can act before any damage is done. Diagnostics based on existing field data streams can be applied to any LIB system without the need for any product modification. The concept of cloud-based battery analytics is presented in Figure 1 and summarized in the following.
- Step 1: Data acquisition. The starting point for all cloud analytics is the continuous stream of measurements from the BMS (“raw data”). This raw data is passed to the communication bus and then pushed to the cloud where it can be stored, consolidated, and analysed by the battery operator or a third-party service provider.
- Step 2: Data pre-processing. To leverage the raw data, extensive data cleaning needs to be performed. For one, outliers and systematic measurement errors in the raw data need to be detected and flagged as such, to avoid false interpretations. But, more generally speaking, every BMS has its own systematic and statistical errors and idiosyncrasies that need to be understood to make sense of the data. If you plan to evaluate a cloud-based analytics solution, then make sure to do a proof of concept to ensure it works with any kind of input data and is able draw the right conclusions from every new data point. This pays off in terms of scalability for diverse BMS’s.
- Step 3: Fault detection. Fault detection algorithms scrutinize the battery data to check for potential faults. A fault can be identified through changes in primary parameters such as voltage, temperature, and current or in secondary parameters such as impedance, a shift in the open circuit voltage curve, or the amount of active lithium in each cell. To track secondary parameters, model-based algorithms are used, which consider reduced order physical and chemical processes through mathematical equations. Identifying and tracking specific patterns in these parameters from millions of similar cells, which are in operation, enables these algorithms to find anomalies before they become dangerous.
- Step 4: Reporting. If a battery is identified as dangerous by the battery analytics, automated warning notifications are generated so the owner can take precautionary measures and arrange for maintenance or replacement.
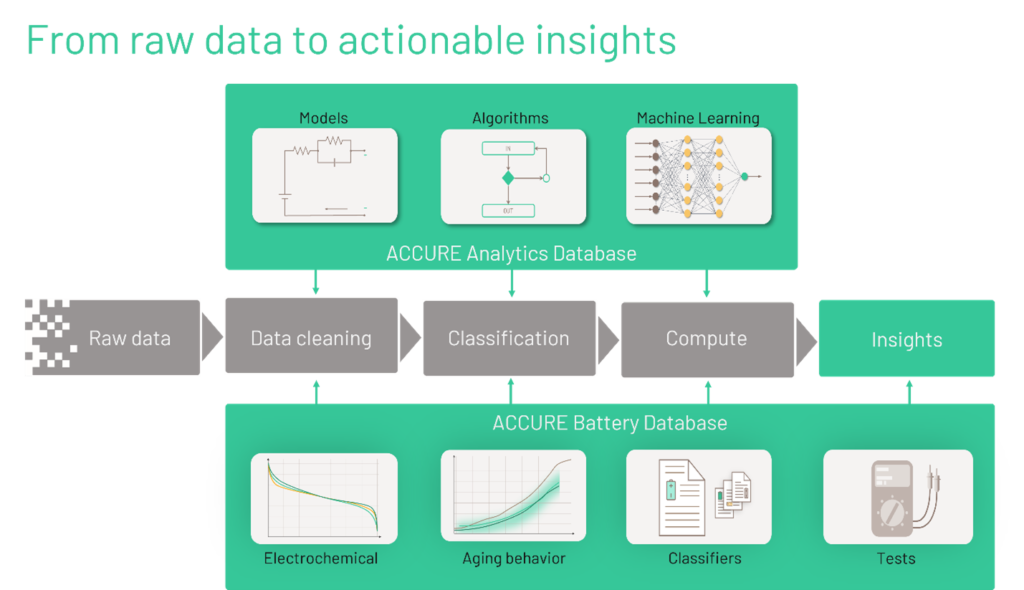
Figure 1: Schematic workflow of ACCURE’s predictive battery diagnostics
A Technical Example of One of 20 Safety Indicators
There are many ways field data can reveal safety-critical battery behaviour long before the BMS does. In fact, there are at least 20 safety indicators a robust battery analytics solution should track daily. They are based on electrical, thermal and mechanistic models empowered by machine learning. The algorithms mirror electrochemical relationships and processes, revealing insights about the internal states of the battery. In Figure 2, an analysis of the loss of lithium inventory, a process closely linked to lithium plating, is presented.
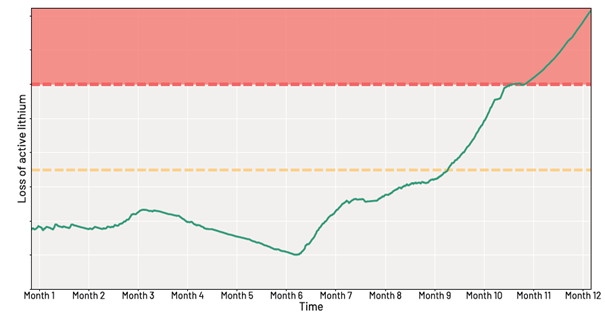
Lithium plating, where metallic lithium gathers on the outside of the anode, has been a major headache in the LIB world for decades. It mainly occurs when a battery is charged with high current rates at low temperatures but can also happen under “normal” operating conditions. Not only does it quickly degrade a battery’s capacity, but it can also become a safety threat by forming metallic dendrites and triggering side reactions such as gassing. It manifests itself in a decrease of the lithium inventory which is no longer available for the main reaction. Cloud-based safety algorithms, among other things, must closely track the loss of active lithium to accurately predict safety critical events.
Safety Monitoring with Cloud-Based Battery Analytics
At ACCURE Battery Intelligence a team of 70 battery experts applies cloud analytics to optimize the safety, reliability, and lifetime of currently over a half million battery packs in mobility and energy applications. Our battery analytics solution Safety Manager has already prevented more than 50 fires since 2021. Among our customers are companies that have experienced battery fires and other safety incidents, which are not often publicized, before coming to us. One public example, however, is the Berlin Transport Authority (BVG) who will transition their entire fleet to electric vehicles by 2030 – a total of 1,600 buses. After several battery fires in e-buses across Europe earlier this year, BVG decided to be proactive about safety and started to use ACCURE’s cloud-based safety monitoring on a part of their fleet.
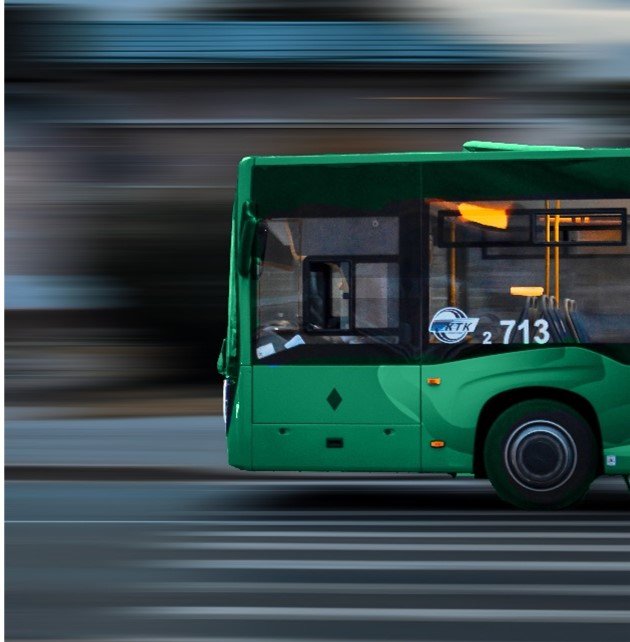
There are many hurdles we need to overcome for electric vehicles to substantially replace ICEs. Cloud-based battery analytics ensures that public opinion about EV safety does not become one more hurdle to overcome. Instead, the benefits of software analysing operational battery data extend beyond an additional safety layer and into reducing business risk and supply chain costs, accelerating innovation, and increasing the sustainability of batteries. There’s a lot more to come out of this exciting field!
About the author
Dr. Kai-Philipp Kairies is a scientist and entrepreneur focusing on innovative storage solutions. He worked as a battery researcher and consultant in Germany, Singapore, and California.
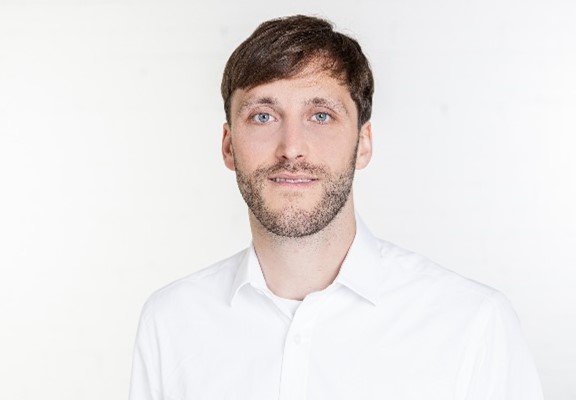
Since 2020, he is CEO of ACCURE Battery Intelligence, a research-lead software company that supports electric vehicle manufacturers, electrification project managers, and fleet managers in understanding and improving their batteries’ safety, longevity, and profitability using advanced, cloud-based data analytics.