Reducing the risk of battery fires with functionally enhanced venting units
Dr. Michael Harenbrock
As the automotive market recovers from the pandemic, we observe an acceleration of electric vehicle market penetration. This is supported by governmental policies and subsidies stimulating the industry, but also driven by increased customer interest as significant technical improvements have been achieved in battery technology. For example, the energy density on cell level has almost tripled in the last ten years, leading to electric driving ranges more and more customers find acceptable.
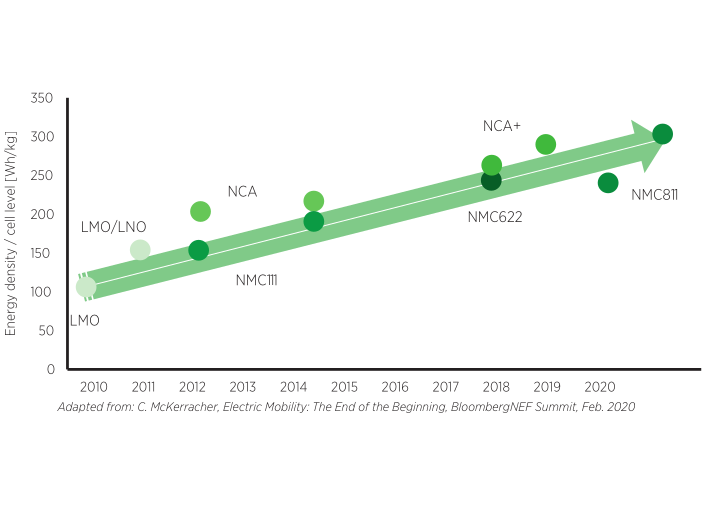
With much more energy stored inside a high-voltage battery pack, the higher amount of energy released during thermal cell or pack failures can lead to more severe consequences, e.g. battery fires. As a consequence, the need to implement safety features to prevent thermal runaways and resulting battery fires is essential to protect drivers and passengers from any harm, and to build customer trust and confidence in this technology. But it will never be completely possible to rule out the risk of thermal runaways completely – so at least the negative impacts they have on vehicle occupants must be minimized by a comprehensive safety strategy.
This need is also reflected in international regulations, e.g. in article 5.4.12.1 of UN GTR 20, “Global Technical Regulation on the Electric Vehicle Safety (EVS)” and article 5.2.7 b of Chinese GB 38031-2020, “Electric Vehicles Traction Battery Safety requirements”. The aim of both regulations is to give passengers at least five minutes to safely leave a vehicle after the first battery cell goes into thermal runaway and triggers thermal propagation by heat transfer to adjacent battery cells:
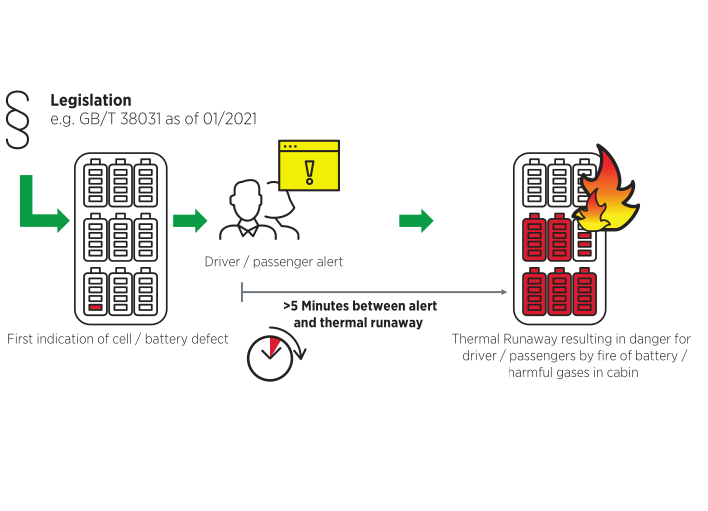
UN GTR 20: “The vehicle shall provide an advance warning indication to allow egress or 5 minutes prior to the presence of a hazardous situation inside the passenger compartment caused by thermal propagation which is triggered by an internal short circuit leading to a single cell thermal runaway such as fire, explosion or smoke”.
GB 38031-2020: “In accordance with 8.2.7.2, conduct thermal propagation occupant protection analysis and verification. 5 min before a danger is caused in the passenger compartment due to thermal propagation, as a result of thermal runaway of a single battery, …, and the safety requirements shall still comply with 5.1”.
The course of a thermal runaway event strongly depends on factors like cathode chemistry, state-of-charge, cell size, trigger method etc., but typically consists of two phases – phase 1 dominated by electrolyte- and interface-related reactions with limited heat generation and venting of some gas, e.g. evaporated electrolyte and its decomposition products. Melting of the separator leads to internal shorts and thus the highly exothermic phase 2 with the major release of heat, gas and particles, and propagation to adjacent cells.
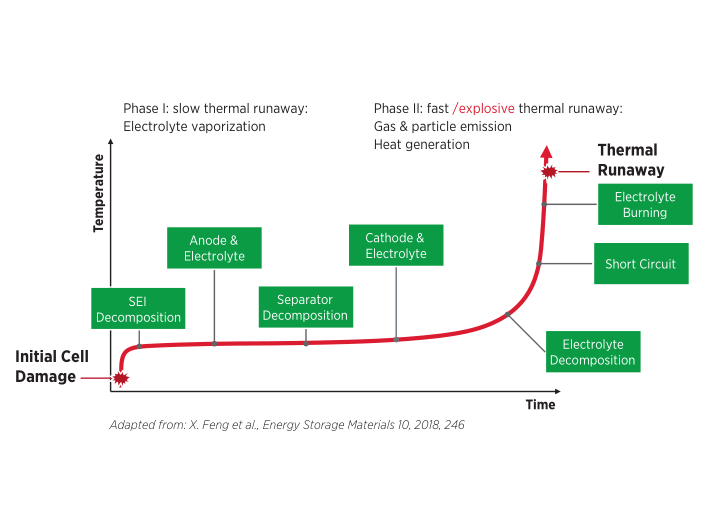
Protection strategies must address all three battery levels: cell, module, and pack. On cell level, quality control in cell manufacturing must prevent e.g. contamination by particles inside the cell, while battery management ensures the balanced charge and discharge of individual cells to avoid high stresses. On module level, the focus is on prevention of thermal runaway propagation, e.g. by using insulating barrier layers between cells. On pack level, measures are included to reduce the risk to passengers caused by a thermal runaway, e.g. by integration of burst discs which release vent gases fast and in a controlled manner to the environment which is the main focus of this article.
In thermal runaway events, large volumes of vent gas are created in a short period of time which leads to a steep increase of pressure inside the battery pack. So devices must be integrated into the pack wall which enable fast and controlled release of gases to limit the massive pressure build-up inside the pack. One way to achieve this is to add a burst disc functionality to a venting unit which enables pressure balancing between battery pack interior and environment. In the design shown in figure 4, the white, semi-permeable PTFE membrane allows pressure balancing under standard operating conditions.
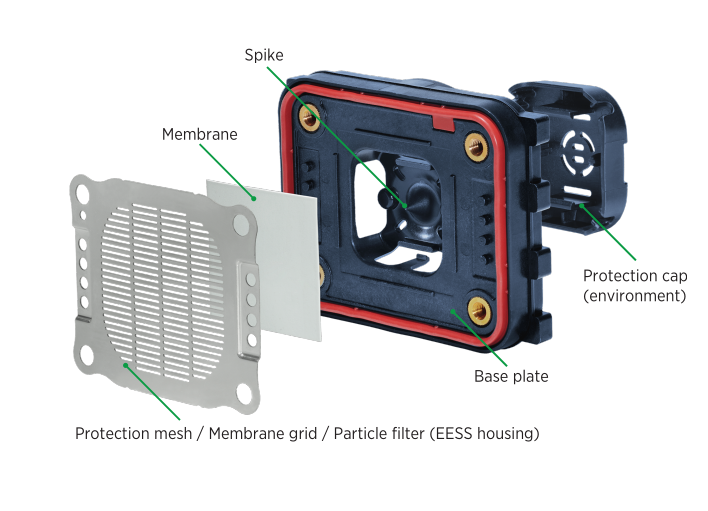
Being chemically stable and hydro- as well as oleophobic enables lifetime applications for automotive use, achieving ISO 20653 protection class IP6k9k. The spike, integrated into the base plate, makes the membrane function as a burst disc: if the pressure inside the pack rises over the pre-defined level, the membrane is pushed against the spike which ruptures the membrane in approximately 100 to 200 milliseconds, opening a large area through which the vent gas can exit the pack fast.
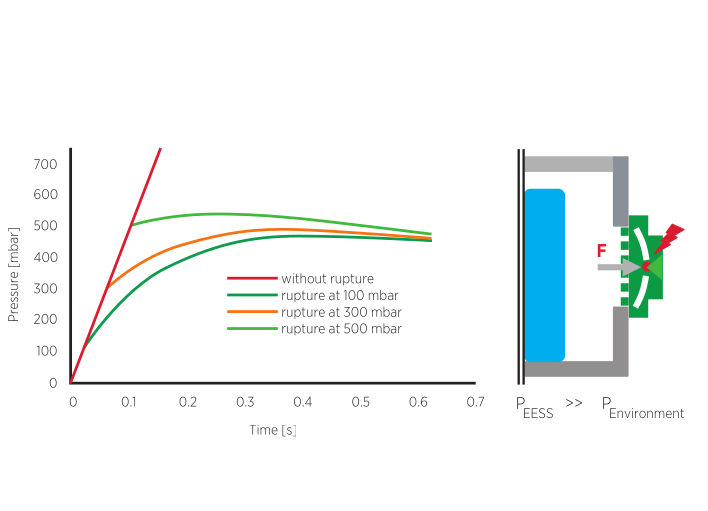
By proper placement of the unit, the vent gases can be guided away from potentially critical positions like the cabin.
Another challenge is to reduce the risk of battery fires after thermal runaway. A fire is an exothermic, uncontrolled reaction between a flammable gas and oxygen, e.g. from ambient air. In addition, an energy source, for example a spark, is required to ignite this mixture. But this only happens if oxygen and flammable gas concentrations are inside the ignition limit range. In a thermal runaway event, a complex gas mixture is created. The oxygen content inside the pack can be too low to create a flammable gas / oxygen mixture. But as it exits the pack, it gets mixed with ambient air which increase the oxygen concentration, so hot particles ejected from the battery pack can act as sparks, igniting the gases outside of the pack.
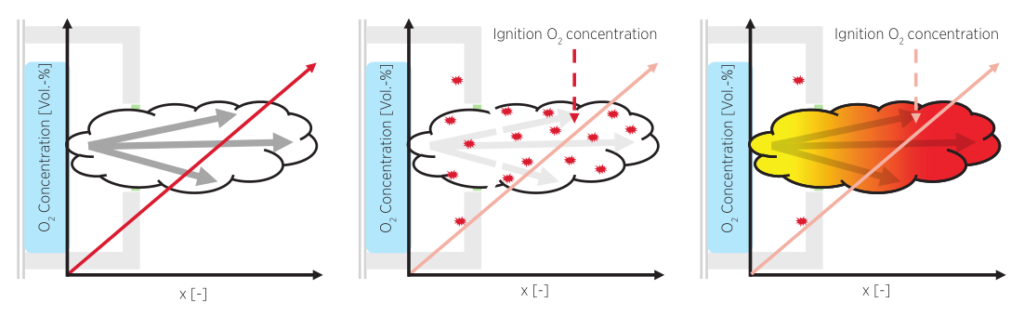
One way to prevent this from happening is to add a filter which will keep those particles inside the pack, thus reducing the risk of ignition.
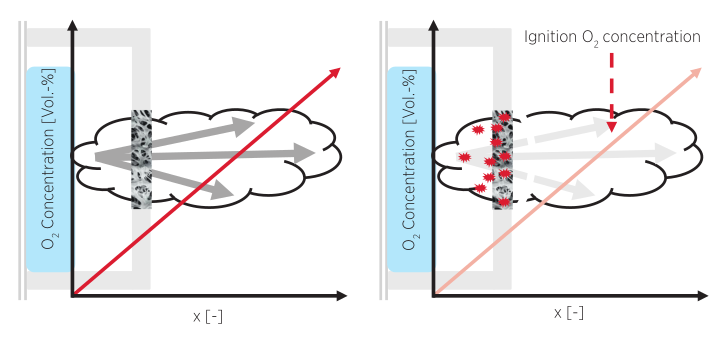
To properly design such a feature, two questions must be answered: which particle sizes must be filtered, and how much particle mass must be captured? During thermal runaway, particles in different size classes are released – from nano-scale soot to particles from electrode material to metal globules created by melting metal components to millimetre-sized shreds from battery cell cans and current collectors. If a particle can ignite a gas mixture or not depends on the energy it carries, which depends on particle mass and its heat capacity. Fig. 8 shows results from particle analysis after controlled thermal runaway tests with Ni-rich NMC-cells. More than 50% of captured particle mass contained particles with a diameter of 0.85 mm and above, giving orientation for filter design.
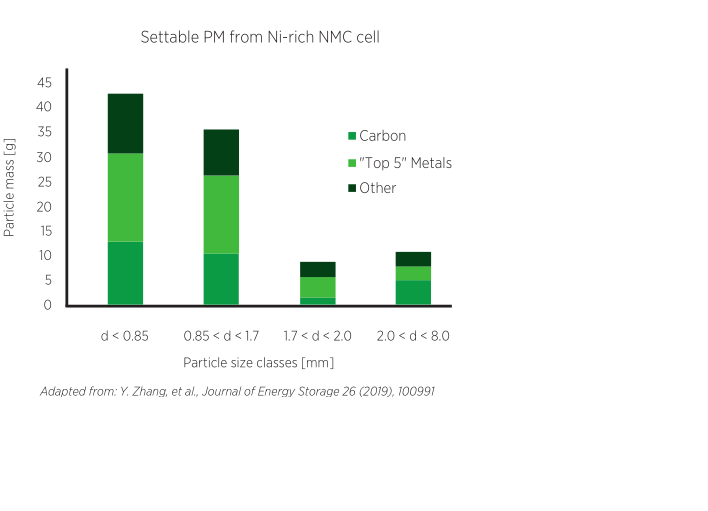
Of course, as many particles as possible should be captured – not only to reduce the risk of battery fires, but also to remove health-relevant particle emissions. The two design concepts contain an additional metal grid to capture the particles. Depending on requirements on integration into the pack, the additional filter can either be inside or outside the pack.
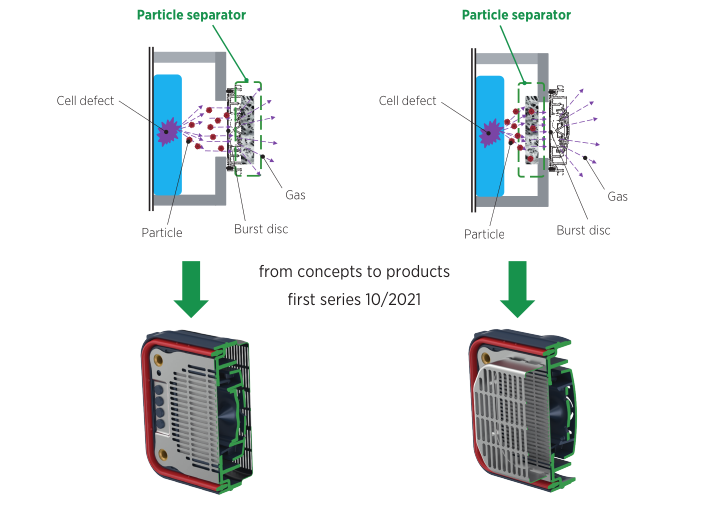
With this, let´s take a look into the future – so what´s next? Sensors might enable early warning for the vehicle´s occupants. Recent work shows that gas sensors might be a good option for this. The sensors should work with all battery chemistries and state-of-charge levels, have a strong signal even at low gas concentrations, show no cross-sensitivities, and be stable under typical automotive operation conditions. Considering results from controlled thermal runaway events with different cell chemistries and state-of-charges, CO2– and H2-sensors seem to be promising candidates.
Thermal runaway events and thermal propagation can never be completely ruled out, especially as larger and more energy-dense high-voltage battery packs are going to market. So appropriate safety measures on all three levels – cell, module, pack – must be integrated to realize a holistic safety strategy for battery electric vehicles. This is a prerequisite for customer trust, confidence and acceptance of battery electric vehicles.
Dr. Michael Harenbrock, Principal Expert Electric Mobility, MANN+HUMMEL