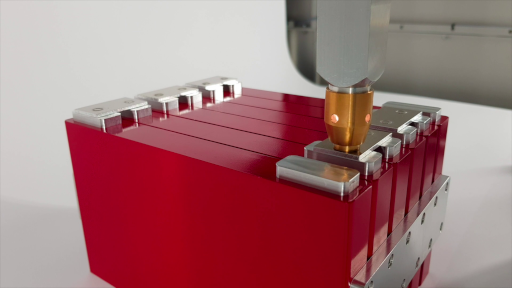
We are in the midst of a veritable technological revolution: a switch to battery power. E-Vehicles are the most widely discussed phenomenon, but the same exuberant growth is happening in more mundane fields, such as power tools, lawnmowers and stationary energy storage.
Whether a small, hand-held power pack or a battery module for a city bus weighing several tons, an enormous range of different batteries shares a common basic element, i.e. the Li-ion battery cell. Again there are many different sub-species of cells all designed for specific duty profiles, but as concerns the mechanical build-up, there are really only three major types: prismatic cells shaped like a cigarette box or paperback; pouch cells, also known as coffee-bag cells; and small cylindrical cells such as the 18650 or 21700 type popularized not least in the e-vehicles from Tesla Motors.
No matter what the final battery looks like, it is always made up by an assembly of identical cells, ranging from just a few to many thousands. They all have to be wired together electrically, and of course they need some sort of housing package for protection and to allow efficient handling.
Not surprisingly, there is quite a zoo of interconnection technologies available, each with its particular profile of strengths and weaknesses; not surprisingly, what’s making them different are often issues of cost and quality. There is also an added complexity caused by the fast growth of the market: any new application may turn into a huge commercial success, requiring a fast scale-up of the production volume in order to gain or keep market share. So time-to-market can be of especial importance, and what’s good for small production volumes may be a drawback once the volumes grow.
One way to group the interconnection technologies follows the amount of current that is carried between cells. For small cylindrical cells, these typically run in the range of 10 to 20 A and are often handled by Nickel connectors made out of thin sheet metal and applied by resistance welding. This process is widely used particularly for new entrants; simple to introduce at moderate cost if a manual welding station is used; and can be scaled up with automatic equipment. Against this, there are two major weaknesses: the welding quality is not always perfect and hard to monitor during the process, leading to inexplicable yield losses for random batches; and secondly, the process is not straightforward to ramp up because the automatic equipment version is completely different.
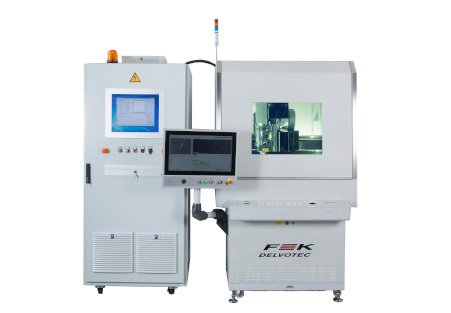
The other connection process which is popular for cylindrical cells is ultrasonic wire-bonding. It has been around for decades in the semiconductor industry and enjoys the advantage of being a cold-welding process, i.e. it produces a true bulk connection but without a molten phase, hence it is much gentler on the thin metal cell containers. The bonding quality is extremely high at practically 100% yield; and excellent process control tools exist to monitor shifts in surface quality and the like. A drawback is that the entry-level equipment is considerably more expensive than for resistance welding; however, scale-up is very easy because automatic parts handling can be added later while the basic equipment stays the same, removing training and switchover troubles. Not surprisingly, ultrasonic wire-bonding has become the leader in volume ever since very large producers such as Tesla adopted this technology.
Once currents go beyond 40 to 50 A, the limits of wire-bonding become troublesome because a single aluminium wire can only carry around 20 A before fusing. Bonding multiple wires side-by-side is feasible but not really cost-efficient, and ribbons instead of wires are difficult to process because of the much higher ultrasonic energy involved and hence the trouble to keep parts firmly fixed in place against the mechanical oscillations.
There and even more so for prismatic cells where currents of around 200 A have to be conducted, Laser welding and Laser bonding come into play. Connectors with large cross-sections are employed, and those are most frequently processed by laser welding. The trouble with such connectors is that they are typically tailored for a particular cell layout. Hence there are substantial tooling costs, making prototype and pilot manufacture cumbersome and slow.
Laser bonding, a newly developed technology from a collaborative project by Fraunhofer ILT and F&K DELVOTEC, is an excellent alternative because it uses a copper or aluminium ribbon that is drawn off a spool and bonded from one connection point to next before being cut. Therefore any length of ribbon can be placed in any position or direction. Moreover, the welding head always pushes the joint down onto the cell pole, compensating height variations from cell to cell. Another advantage of this process is that it is not sensitive to surface contaminations, and it requires minimal clamping to keep the cells in place.
Ramp-up is a particularly strong point of Laser Bonding: for large production volumes of a given layout, connector plates or masks tailored to that particular battery module can be used, just like in a conventional laser welding setup. In this mode, the laser bonder only has to make a single connection point per cell pole and hence is more productive. Given that the very same laser bonder can be used for both processes, scale-up is extremely easy, and both processes can even be run alternately. Being able to intersperse a quick prototype or pilot run into large-volume production is a huge advantage in this fast-moving industry segment.
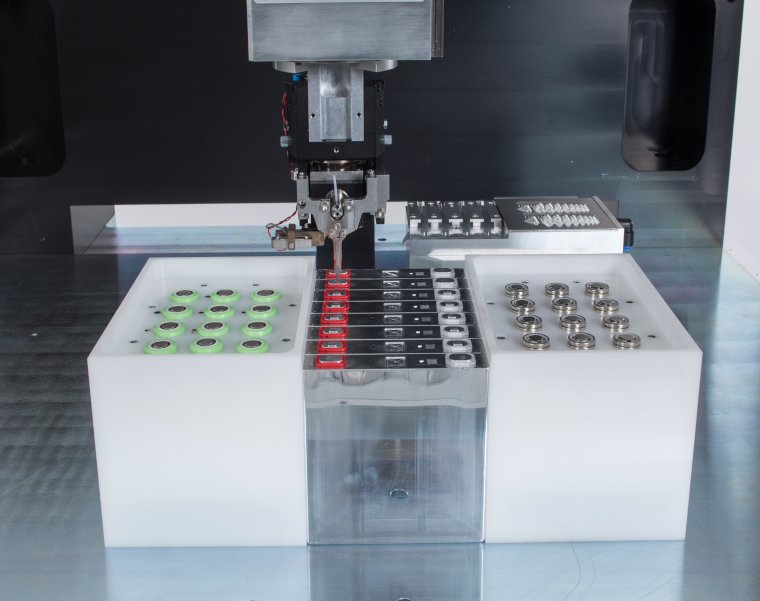
For the third cell type, pouch cells, stacks of aluminium or copper foil tabs have to be welded together within a pouch during cell manufacture. For the battery pack, the resulting outer connection tab has to be connected to a busbar. Given the high currents of 200 A and beyond, laser or high-powered ultrasonic welding is the preferred method. Ultrasonic welding is relatively cheap but suffers from the risk of mechanical interference between bonding steps, damaging the first stack of welds when the second weld is made. Laser welding is therefore gaining ground even though the initial investment for the equipment is higher. At F&K DELVOTEC we are working to implement the advantages of the Laser Bonder also in this particular field.
Finally, there are conventional interconnection technologies like screw-and-bolt, clamping or spring-loaded contacts to provide the connections between cells. They all suffer from the drawback that they are not continuous bulk connects, so they are prone to interface corrosion and increasing electrical resistance, however slow. At the lifetimes of many years required in e-mobility, this is not acceptable for most applications.
Taken all together, there are large uncertainties as to which direction the developments in battery technology will take, making it all the more important to focus on technologies providing both high growth potential, i.e. easy scale-up, and outstanding product quality. Depending on which cell type one is focused on – and it is by no means certain which one of the three major contenders will ultimately prevail – it looks like ultrasonic wire bonding and laser bonding will be staying ahead of the pack.
Author:
Dr. Josef Sedlmair, F&K DELVOTEC Bondtechnik GmbH, Ottobrunn /Germany