Application of MWCNTs to Improve the Performance and the Processability of LIB Electrodes:
Hamidreza Beheshti & Dr. Alicia Rul
Electromobility has become inevitable. Passing environmental regulations like the COP21 Paris accord to combat climate change has already drawn a clear roadmap toward energy transition. On the other side, the growing trend in Electric vehicle (EV) adoption pushes the automotive industry to shift from fuel-running to electricity-powered vehicles. The development of EVs requires battery supplies and accordingly, market studies predict a constant growth in battery production capacity in the upcoming years. Forecasts for a fivefold increase in the annual cell production capacity from 2020 to 2030 illustrate the potential as well as the challenge ahead. Li-ion Batteries have currently conquered the industry due to their many advantages, namely high voltage and capacity plus their well-established production and value chain. However, the mass adoption of EVs demands improvements in several aspects. Extending the driving range, increasing the charging rate, diminishing the safety concerns, and reducing the prices could be mentioned as the most appealing factors to expand the EV market. However, improving vehicles’ efficiency and reinforcing the charging infrastructure pave this way, breakthroughs are essential in cell performance. Such advancements require improvements at the material level. Innovations in battery materials are not only an approach to enhance the electrochemical performance such as energy and power output, but also they could affect the cost-effectiveness, manufacturability, and safety of LIB cells. The advanced battery materials therefore should offer an enfolding package of all these features.
Conductive carbon is a basic component of electrode slurries. Multi-Wall Carbon Nano-Tube (MWCNT) is one of the materials that could be used for that purpose. This novel material features a set of exceptional properties that turn it into the additive of choice for advanced lithium and Li-ion batteries. Figure 1 compares some of these characteristics between MWCNT and some of the other carbonous compounds. When compared to the conventional carbons applied as electrode additives, MWCNT features a much higher surface area (at least around 4 times) elevating its electron-conducting characteristics. From the other aspect, MWCNT features much higher tensile strength values than rudimentary carbons like Carbon Black (CB) for about 20-120 times and additionally makes a 3D network reinforcing the matrix in which the active material particles are distributed. Such properties become even more important in the development of Si-based anodes. Despite their superior capacity, Si anodes suffer from several drawbacks such as a large volume change of about 400% during the (de)intercalation process causing pulverization of the electrodes and instability of the solid electrolyte interphase (SEI). The poor intrinsic electrical conductivity of Si-based materials on another side negatively affects the electrode kinetics, particularly at higher cycling rates. The application of MWCNT forms a conductive and flexible network that addresses these issues toward the development of Si-based anodes.
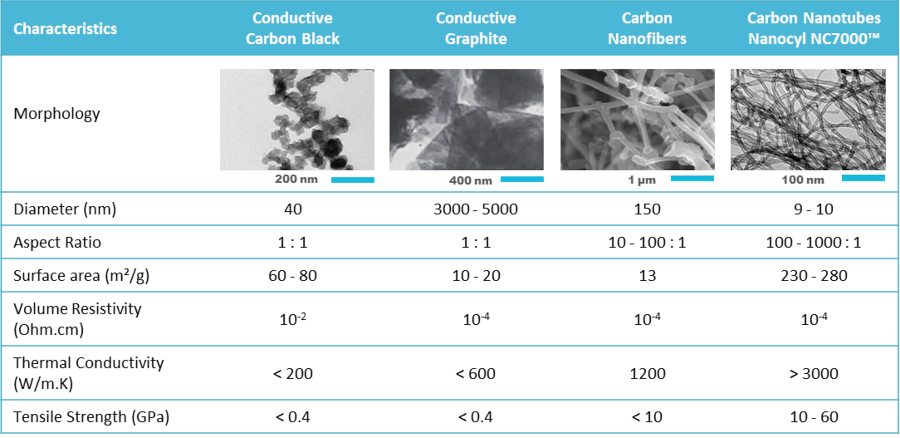
Figure 1. Comparison of intrinsic properties of the main LIB conductive additives
The other advantage of MWCNT over conventional carbon compounds comes from the perspective of energy density. As a result of a higher active surface area and increased conductivity, a lower mass of MWCNT could provide the same yet more electrical conductivity to the system than the additives like CB. Reducing the total amount of carbon additive while reaching the target conductivity increases the active material contained in electrode formulation leading to cells with an increased capacity and energy density. Figure 2 presents the percolation curves of different conductive additives in LCO cathode films with 2% of PVDF binder.
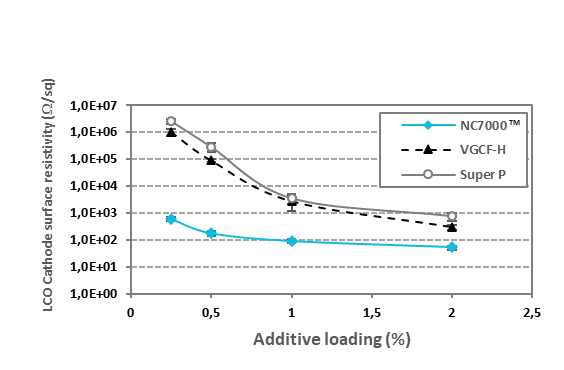
Figure 2. Percolation curves in LCO cathode films with 2% PVDF binder
MWCNT could be used in synergy with carbon black as well. This approach not only ameliorates the surface conductivity but also provides a more homogenous charge distribution, as demonstrated in Figure 3.
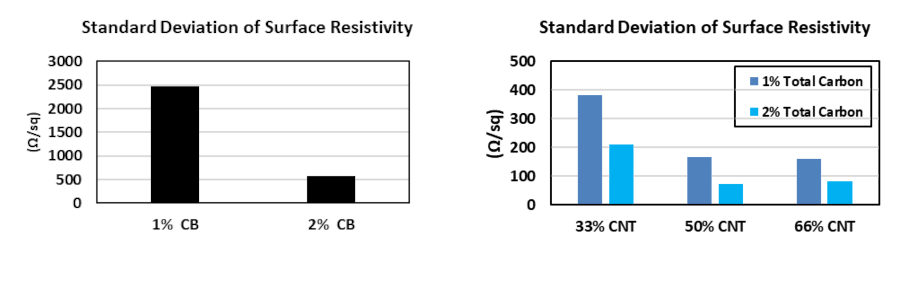
Figure 3. Comparing the homogeneity of the electrical conduction network in the NMC electrodes with CB (left) and CB+NC7000TM Synergy (right)
Regarding its geometrical shape and higher aspect ratios compared to the other carbonous additives (100-1000 times), MWCNT can modify the morphology of the electrode structure which could be considered as an approach to improve the rate capability. Reaching a good balance between energy and power is a challenge. Depending on the cell design, controlling the electrode porosity is an approach to improve the particle wettability and ion transportation toward higher power output. Contrary to CB, the MWCNT structure provides more flexibility and process adjustability to tune the morphology during electrode processing and achieve a better balance between energy and power if needed. Together with increasing the energy density via decreasing the required additive loading, electrode porosity could be tuned as well with lower MWCNT content compared to conventional carbon black as presented in Figure 4.
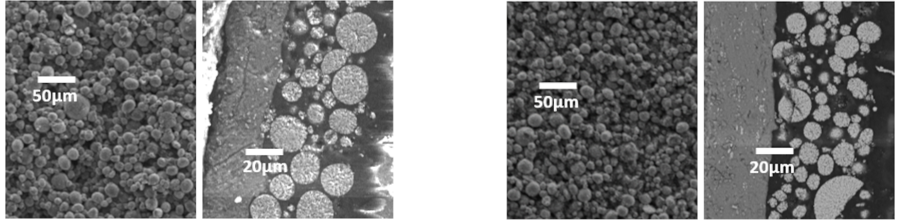
Figure 4. The Cross-sectional SEM photos of NMC Cathodes comparing the porosity of the films containing 1% of NC7000™ MWCNT (left) and 3% of conventional carbon black (right)
Added to many performance upsides, replacing conventional carbons with novel MWCNT facilitates the electrode fabrication process as well. Given the difficult dispersion process required for a homogenous distribution of the carbonous additive in the slurry, reaching conductivity targets with reduced carbon content would assist electrode processing with reduced technical complications and energy consumption. Of course, the rheological properties of the MWCNT dispersion play an important role in its facile adaptability to the slurry preparation process. In that regard, good rheological properties of MWCNT dispersions bring easy handleability to the mixing process and make the process compatible with different types of machinery. The possibility of reducing the viscosity at low shear rates, as presented in Figure 5, is an example of such process compatibilities.
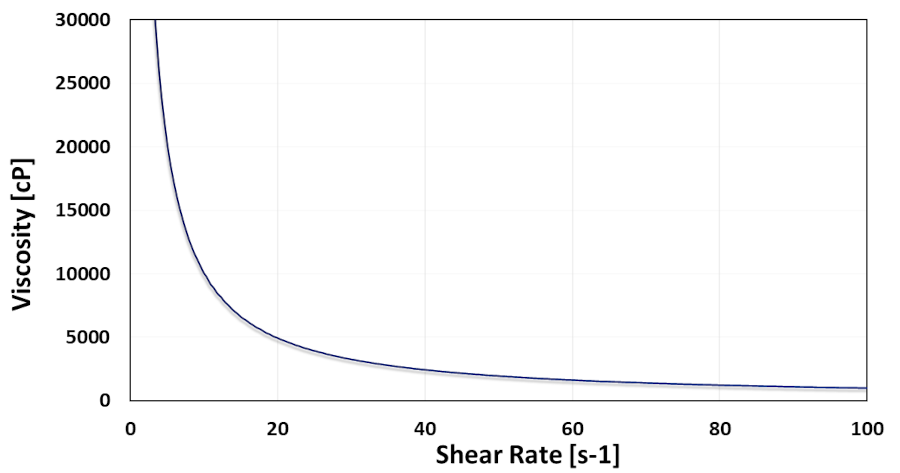
Figure 5. Correlation of viscosity and shear rate in a 4% dispersion of NC7000™ MWCNT in NMP (Orgacyl™)
The result of an appropriate dispersion of MWCNT into the base solvent will be a homogenous electrode with excellent capacity retention even at a high discharge rate and consequently an improved long-term cycle life. Figure 6 depicts the performance of an LFP cathode based on the dispersion of MWCNT into NMP. The discharge rate assessment and the long-term cycling emphasize an extended lifetime with over 2000 cycles with a capacity loss below 10%.
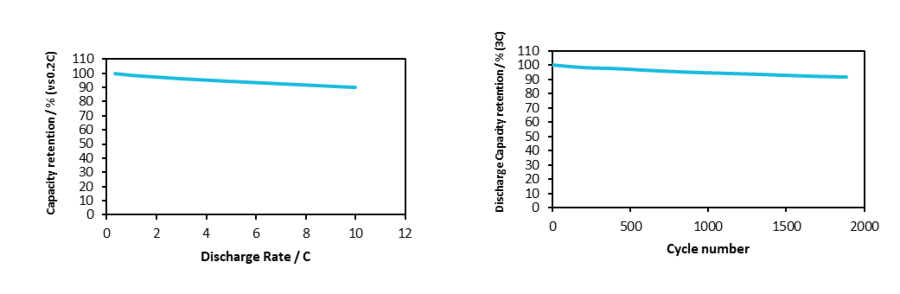
Figure 6. The discharge rate curve (left) and the long-term cycling test (right) of the LFP electrodes based on the dispersion of NC7000™ MWCNT into NMP (Orgacyl™)
Summing up the aforementioned features of MWCNT, these nanotubes offer several electrochemical, electrical, mechanical, and morphological modifications to LIB electrodes. As a result, the application of these advanced additives not only leads to more performant electrodes, but also facilitates the slurry/electrode processing making them efficient, safe, and cost-effective additives for next-generation batteries.
Hamidreza Beheshti, Dr. Alicia Rul, Energy Storage and Liquid dispersion Business Unit, Nanocyl SA